CT03 - CDEV-1 Brutus Buckeye Room (#3044) in The Ohio Union
CDEV Subgroup Contributed Talks
Thursday, July 20 at 2:30pm
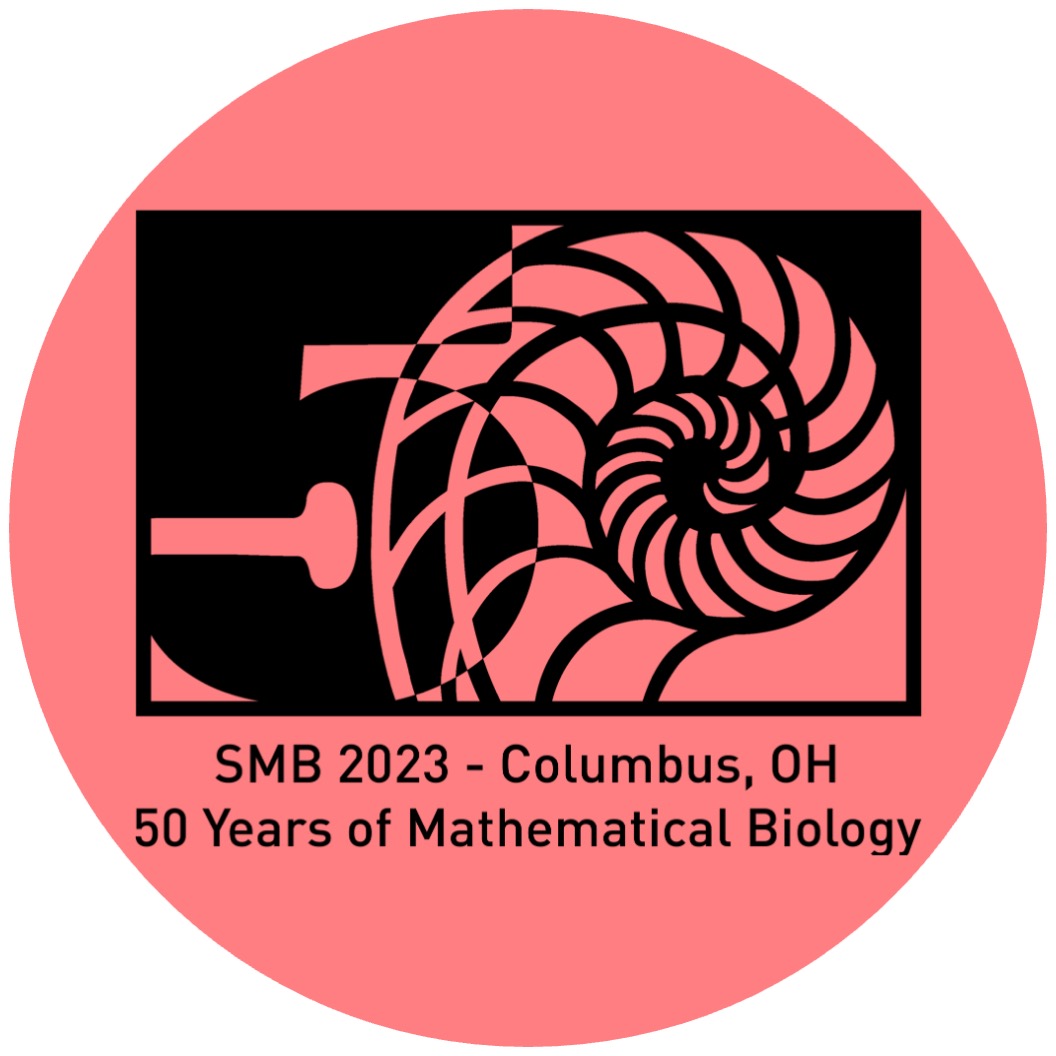
Share this
Adriana Zanca
The University of Melbourne"Comparison of locally and globally acting wound closure mechanisms"
Gordon R. McNicol
University of Glasgow"A one-dimensional continuum model for focal adhesion and ventral stress fibre formation"
Kaiyun Guan
University of North Carolina at Chapel Hill"How do yeast regulate polarity behavior to ensure successful mating?"
Sharon B. Minsuk
Indiana University, Bloomington"Modeling the Mechanical Forces Driving Epithelial Morphogenesis and Cell Rearrangement during Zebrafish Epiboly"
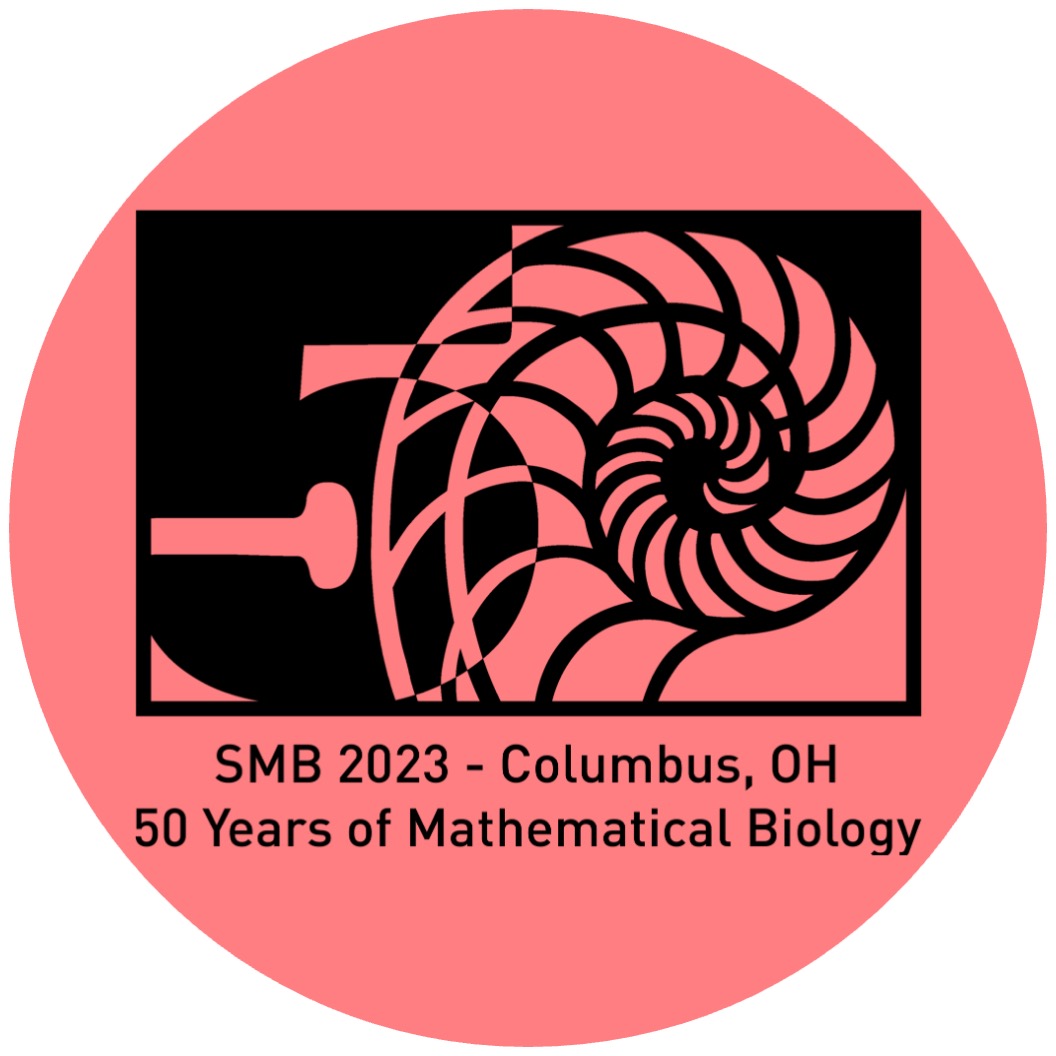