MS04 - CARD-1 Barbie Tootle Room (#3156) in The Ohio Union
Integrating Mathematics Across the Cardiovascular System: A Mini-Symposium on Multilevel Modelling of Cardiovascular Biology
Tuesday, July 18 at 04:00pm
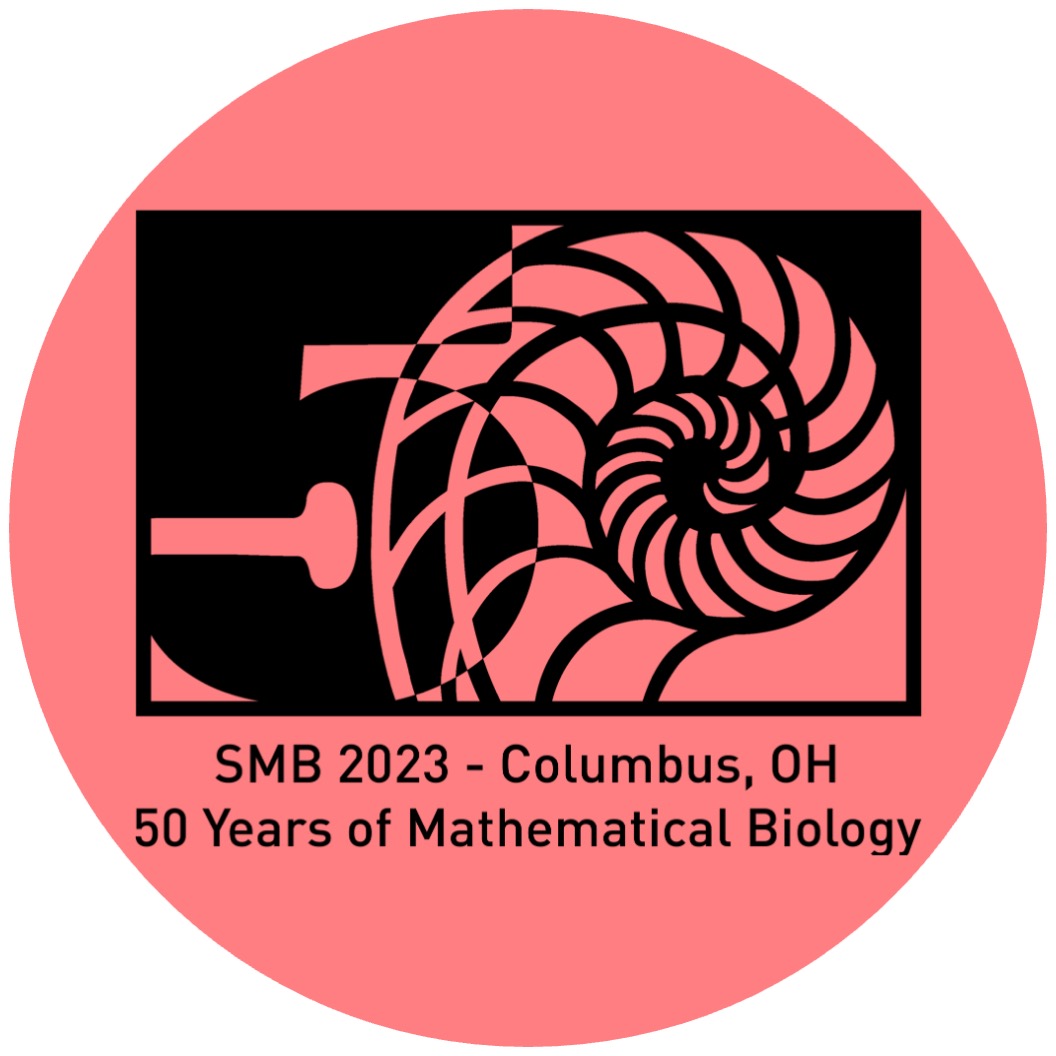
Organizers:
Jessica Crawshaw, Vijay Rajagopal, Michael Watson, Mitchel Colebank, Seth Weinberg
Description:
Cardiovascular biology is a complex and multifaceted field that encompasses a wide range of physical and biological processes occurring across multiple levels, including the cardiac, vascular, and microvascular systems. At each level of the cardiovascular system, mathematical modelling plays a critical role in integrating data and understanding the underlying mechanisms governing these processes. While there has been significant progress in mathematical and computational cardiovascular biology over the past decade, mathematicians and engineers at each level have tended to work in isolated silos, which risks producing a fragmented understanding of the cardiovascular system and threatens to slow scientific progress. This issue carries significant implications for the broader field of mathematical biology, as cardiovascular models frequently serve as building blocks for larger models of other systems, such as developmental biology, oncology, drug development, tissue engineering, and regenerative medicine. To address this challenge, this mini-symposium seeks to provide a forum for collaboration between mathematicians and engineers from each level of the cardiovascular system. The aim is to facilitate the exchange of ideas, foster new collaborations, and promote a more comprehensive understanding of cardiovascular biology through mathematical modelling. The mini-symposium will feature talks by leading researchers in the field covering various topics, including cardiac physiology modelling, vascular disease, microvascular development, and blood flow dynamics. Each talk will showcase innovative mathematical models and computational tools used to understand the complex processes underlying cardiovascular development, function, and dysfunction. This mini-symposium provides a unique opportunity for mathematicians and engineers interested in cardiovascular biology to exchange ideas, discuss recent developments, and foster new collaborations.
Keith Chambers
University of Oxford (Wolfson Centre for Mathematical Biology, Mathematical Institute)"Resolution vs chronic inflammation: A lipid/phenotype dual-structured model for early atherosclerosis"
Alys Clark
University of Auckland, New Zealand (Auckland Bioengineering Institute)"The fetal circulation and its adaption to pathological placental development"
Joyce Lin
Cal Poly State University (Mathematics)"Conduction reserve theory in cardiac tissue with reduced gap junction coupling"
Nicolae Moise
Ohio State University (Biomedical Engineering)"Emergent Pacemaking and Tissue Heterogeneity in a Calcium Feedback Regulatory Model of the Sinoatrial Node"
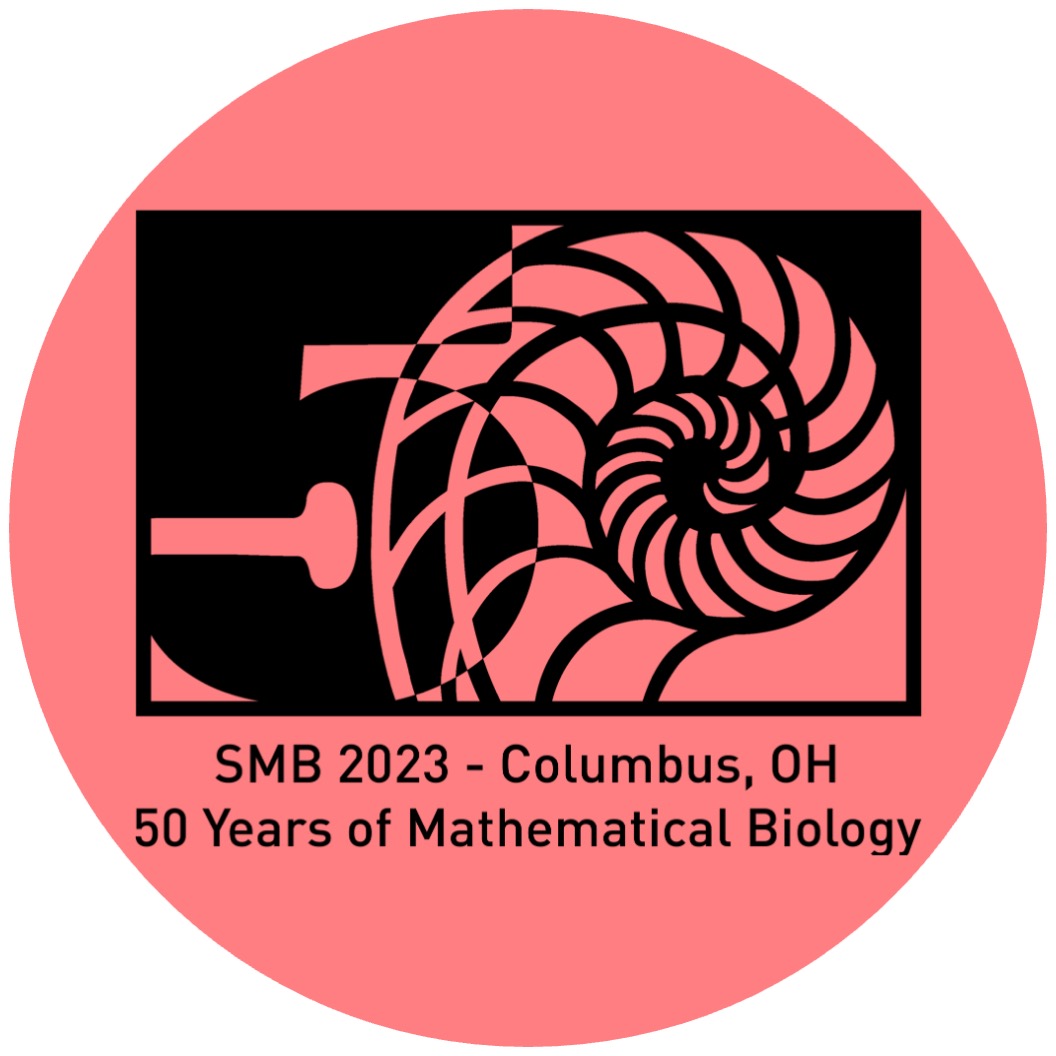